This is the first in series of posts on the role of hydrogen in a low carbon economy. This will look initially at the use of hydrogen as a fuel for road transport, and in particular for cars.
Some General Background
In 2014 UK CO2 emissions were 420 million tonnes. The key emitters were the power industry, general industrial and residential use of combustion, and transport. It has to be remembered however that the power generation industry emits CO2 in order to supply the electrical demand by industrial and residential users.
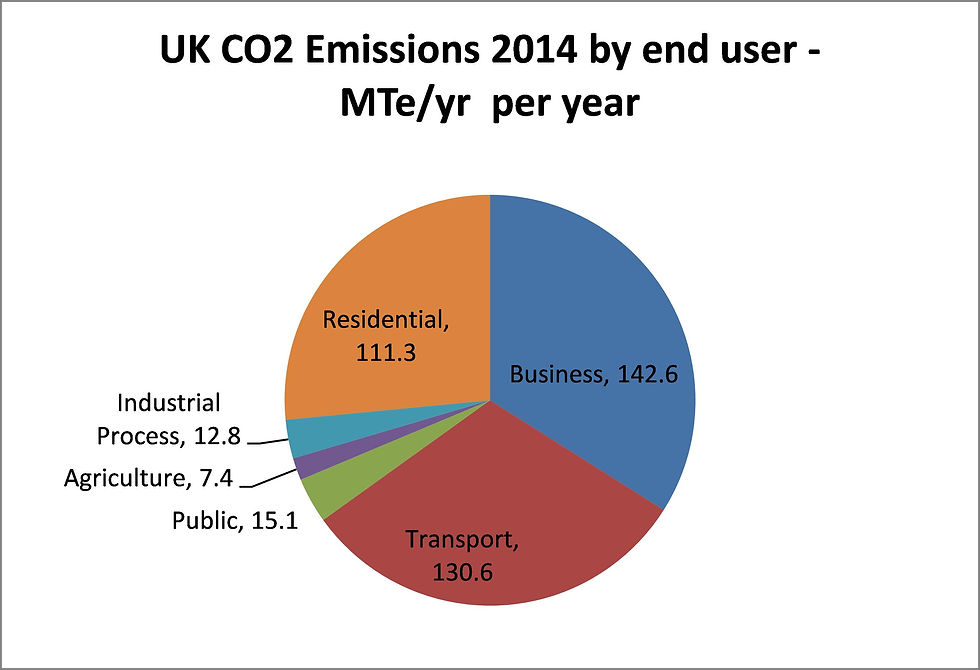
Switching fuel use from natural gas to hydrogen is seen as one possible route to decarbonize both domestic and industrial combustion sources, and a number of studies have been undertaken to evaluate this approach in the UK, for example, the Leeds H21 and HyDeploy studies.
Industrially hydrogen is used for the manufacture of a number of basic chemicals, including ammonia, and where not produced as a by-product is produced almost exclusively from the steam reforming of Natural Gas (NG), producing CO2 as a by-product.
Present UK production of hydrogen is around 683,000 Te per year from some 15 sites. According to the same source roughly half is produced as a by-product, for example in refinery operations and chlor-alkali manufacture. Most is used on site or as a chemical feedstock. The largest use of hydrogen in the UK is in the manufacture of ammonia, in three plants on Teesside, Humberside and at Ince.
Carbon Capture and Storage (CCS) can be applied to conventional hydrogen production to reduce CO2 emissions, but is contingent on long term, stable sequestration of the CO2, either in underground storage as the gas, or mineralized through reaction with suitable naturally occurring minerals to form a stable carbonate.
There are a few opportunities for using CO2 as a feedstock into other processes, such as the manufacture of polyols, where the CO2 is directly incorporated into the product as part of the polymer chain, but such opportunities, whilst valuable in themselves, cannot offer a general solution to the problem of CO2 emissions simply because of the sheer quantity of CO2 released.
The increase in electricity generation from renewables brings with it the growing problem of demand/supply matching. In simple terms, it is difficult to store electricity. The only direct storage method for electrical charge is in super-capacitors, otherwise the electrical energy has to be converted into and held in another form, whether that be as mechanical energy in a flywheel, potential energy in a water reservoir, as heat, as a compressed or liquefied gas, or as chemical energy, as in a battery or other chemical transformation.
One such transformation is the production of hydrogen by electrolysis of water. Hydrogen produced by electrolysis can be stored, then power generated later from the hydrogen using a fuel cell. The overall power-to-power cycle efficiency is relatively low at around 35% or less, but the capital cost is also low. In contrast, battery storage of electricity has a much higher cycle efficiency (75-90%), but is comparatively expensive.
In the context of energy storage, the possibly unique position of hydrogen is that it can also serve as a transport fuel and as a feedstock for chemicals production. The production of hydrogen by electrolysis of water using low carbon electricity, generated either from renewables such as solar and wind, or from nuclear could be a key enabler for the chemical industry and for road and rail transport.
There are a number of other routes for hydrogen production, such as gasification of biomass or waste material such as MSW or RDF (Municipal Sorted Waste, Refuse Derived Fuel). There are potential synergies between gasification and electrolytic hydrogen.
The often proposed manufacture of so-called ‘synthetic’ fuels from CO2 and low carbon derived hydrogen is of questionable viability (on both thermodynamic and environmental grounds) though in some very specific cases there may be an economic or environmental case to be made. For example, in aviation there is presently no real alternative to the use of liquid hydrocarbon fuels. Another very specific example is the manufacture of methanol in Iceland from electrolytic hydrogen (using renewable electricity generated by volcanic heat) and from CO2 recovered from volcanic vents. For Iceland, the driver is to become self-sufficient in liquid fuels, with the country’s energy use based entirely on their own natural resources.
Chemicals and oil processing companies are starting to invest in water electrolysis to meet their hydrogen supply demand, typically based on exploiting the low cost electricity that arises when the output from renewable sources spikes, driving down the market price of electricity.
Germany’s H&R Group started up a 5 MW Siemens PEM electrolyzer in Hamburg-Neuhof in 2017. The hydrogen will be used in refinery processes. It is intended that the plant will exploit low power prices when electricity supply in Germany exceeds demand.
In January 2018 ITM and Shell announced that they will be building a 10MW hydrogen electrolysis plant at Shell’s Rhineland refinery in Wesseling , Germany, scheduled for operation in 2020 (Refhyne project). The plant will have a capacity of 1300 Te of hydrogen per year. Annual hydrogen use at the refinery is 180,000 Te, presently produced by steam reforming of natural gas.
Fuel cells using hydrogen can be used in transport and a number of commercial car models using fuel cells have emerged in the last few years. There are many who question the basic economics and environmental impact of hydrogen fuel cell electric cars (HFCEV’s) compared to battery electric vehicles (BEV’s) and for good reason; the underlying system energy efficiency for a BEV is much higher than that of a HFCEV, whether the hydrogen is made by electrolysis or by steam reforming.
However most have considered the use of hydrogen in transport in isolation from its potential role in energy storage and as an industrial feedstock, and fail to consider exploiting co-produced oxygen.
There is a tendency to offer judgement based solely within the context of private car application, to gloss over issues of range with present BEV vehicles, and to present a false dichotomy where there is a presumption of either BEV’s or HFCEV’s, with no room for any liquid fuel based vehicles including those fuelled by biodiesel or other low carbon fuels.
In truth it is far more likely that the transport fleet will be based on a mixture of fuel types. In their 2018 Global Automotive Executive Survey, KPMG concluded that :
“There will not be a single solitary drivetrain technology: Executives project a similar split by 2040 for Battery Electric Vehicles (BEVs) (26%), Fuel Cell Electric Vehicles (FCEVs) (25%), ICEs (Internal Combustion Engines) (25%) and hybrids (24%).”
Whilst we have passing mention of ICE’s let’s at least put right a common misconception that the UK has banned the sale of ICE vehicles from 2040. It hasn’t. The UK government intends to ban the sale of ‘conventional’ ICE cars; hybrid cars are explicitly excluded. There was also the mis-quoted announcement by Volvo, said in some quarters to be planning to make only electric cars from 2019 – what Volvo did announce is that they would only make electric or hybrid cars from 2019.
The Bossel Chart
A much quoted paper on the subject on the efficiency of BEVs and HFCEV’s is that of Bossel (2006) and his chart from that paper showing the relative system efficiencies is much reproduced, as it is here :
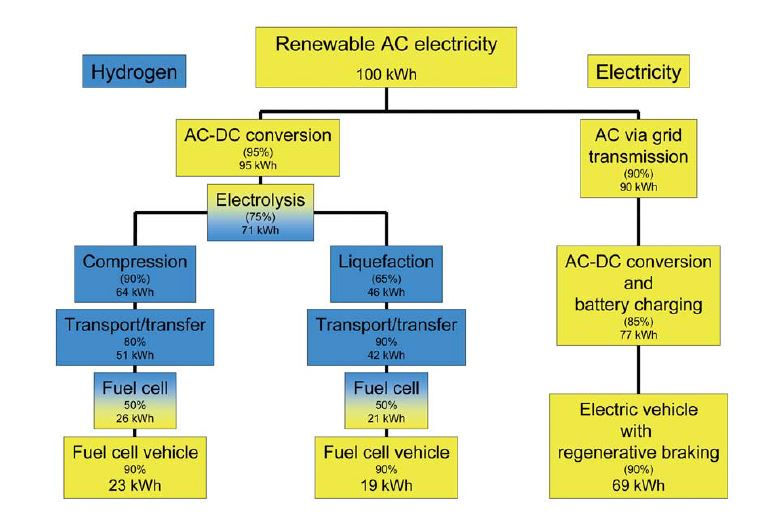
(Bossel Chart)
The chart indicates an overall efficiency for a fuel cell vehicle based on electrolysis hydrogen as a factor of at least 3 lower than for a BEV. Bossel concluded that the energy cost for a fuel cell vehicle would be at least four times that of a BEV, and to the rhetorical title of his paper (‘Does a Hydrogen Economy Make Sense?’) answered ‘Never’. Others have published similar analyses.
There are problems with Bossel’s analysis of the fuel cell based system, in his underlying assumptions and the way in which the calculations have been framed. An independent analysis shows that the power output is a significant under estimate.
Elon Musk, of Tesla and SpaceX fame is quoted as referring to fuel cells (for cars) as ‘mind-bogglingly stupid’, ‘incredibly dumb’ and ‘fool cells’. The technical design director of Jaguar Land Rover, Wolfgang Ziebart, is quoted as labelling hydrogen fuel cell vehicles as ‘complete nonsense’, on the basis of the well to wheel efficiency, though he did concede that there could be some application for hydrogen in energy storage.
However, there is a small but growing number of commercial HFCEV’s available (though only in certain markets) such as the Toyota Mirai, Hyundai IX35 Fuel Cell, Hyundai Nexo and Honda Clarity Fuel Cell. Compared to sales of BEV’, HFCEV sales volumes are low, due at least in part to the lack of hydrogen charging infrastructure.
We will have a look at the Bossel paper and re-evaluate the system efficiency but first we will look at the basics of electrolysis and fuel cell technologies, and, while we are at it take a quick run through some basic thermodynamic principles.
Why do we need to do the latter? The simple answer is that getting to grips with the underlying thermodynamics gives us a sound framework in which to understand the principles behind the energy analysis. It also means that we can clarify and be consistent in the use of terms such as ‘energy’ and ‘efficiency’.
Comments